About
Here, we have generated a combined single-nucleus RNA-sequencing (snRNA-seq) and single-nucleus assay for transposase-accessible chromatin using sequencing (snATAC-seq) NHP brain atlas comprising around two million nuclei from eight brain regions of 13 cynomolgus monkeys (Macaca fascicularis), covering the entire adult lifespan. By integrative analysis of gene expression and chromatin accessibility dynamics, we have investigated the general and region-specific changes in cell type composition, cell state and niche multicellular interactions induced by aging, and the associations of these changes with human genetic risk variants linked to cognition, neurodegeneration, and others. We also highlight key differences between healthy brain aging and AD and uncover potential mechanisms exerting protective effects. Our NHP aging brain cell atlas (NHPABC) and its future expansions will assist in the development of therapeutic strategies aimed at promoting brain health in late life in primates including humans. All raw data have been deposited to CNGB Nucleotide Sequence Archive (accession code: CNP0004459).
Extended Data Figure
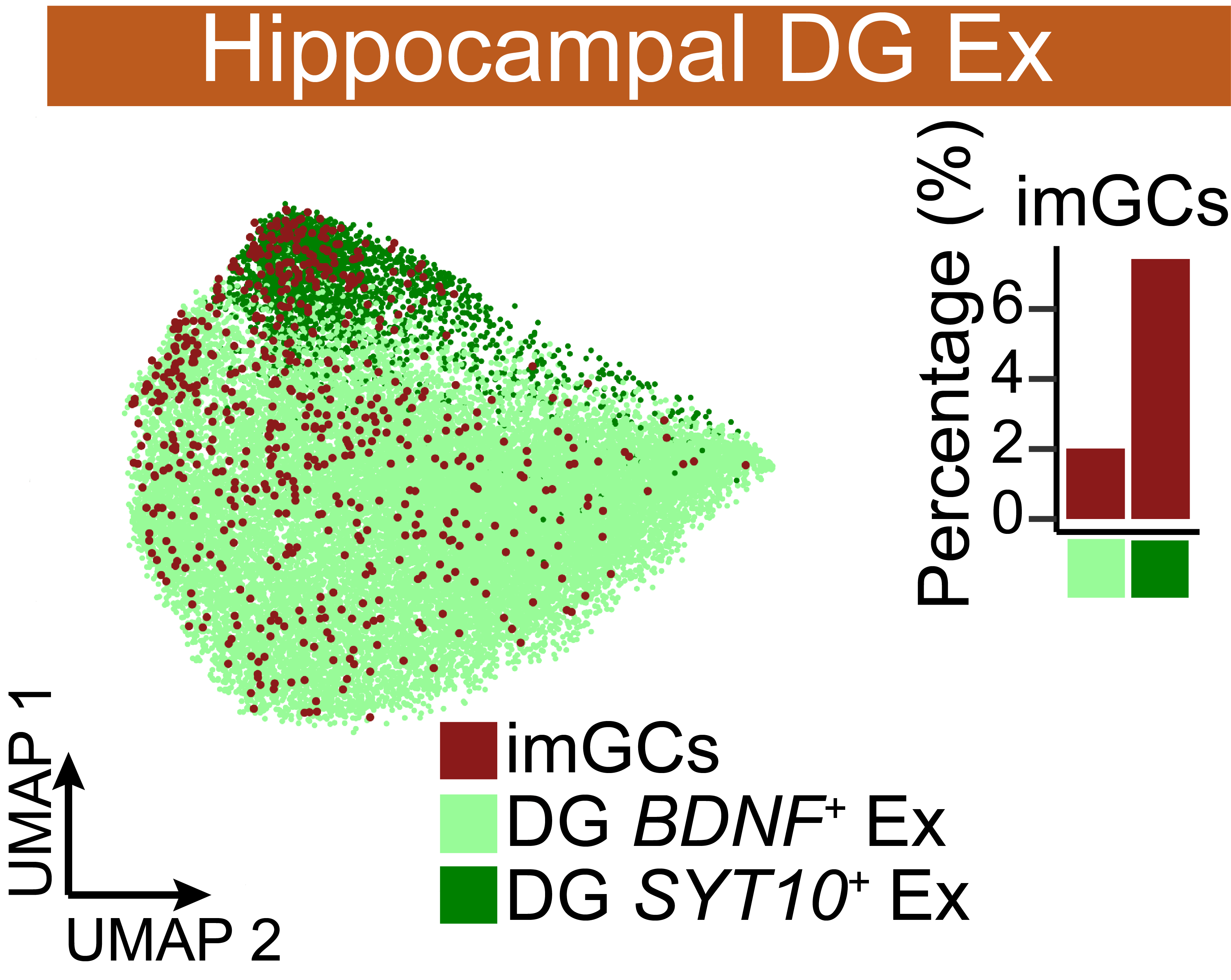
UMAP visualization of reclustered Ex neurons from the dentate gyrus of the hippocampal formation showing the proportion and distribution of immature granule cells (imGCs). The histogram of the right-hand side shows the highest distribution of imGCs within the DG SYT10+ Ex and DG BDNF+ Ex cells.
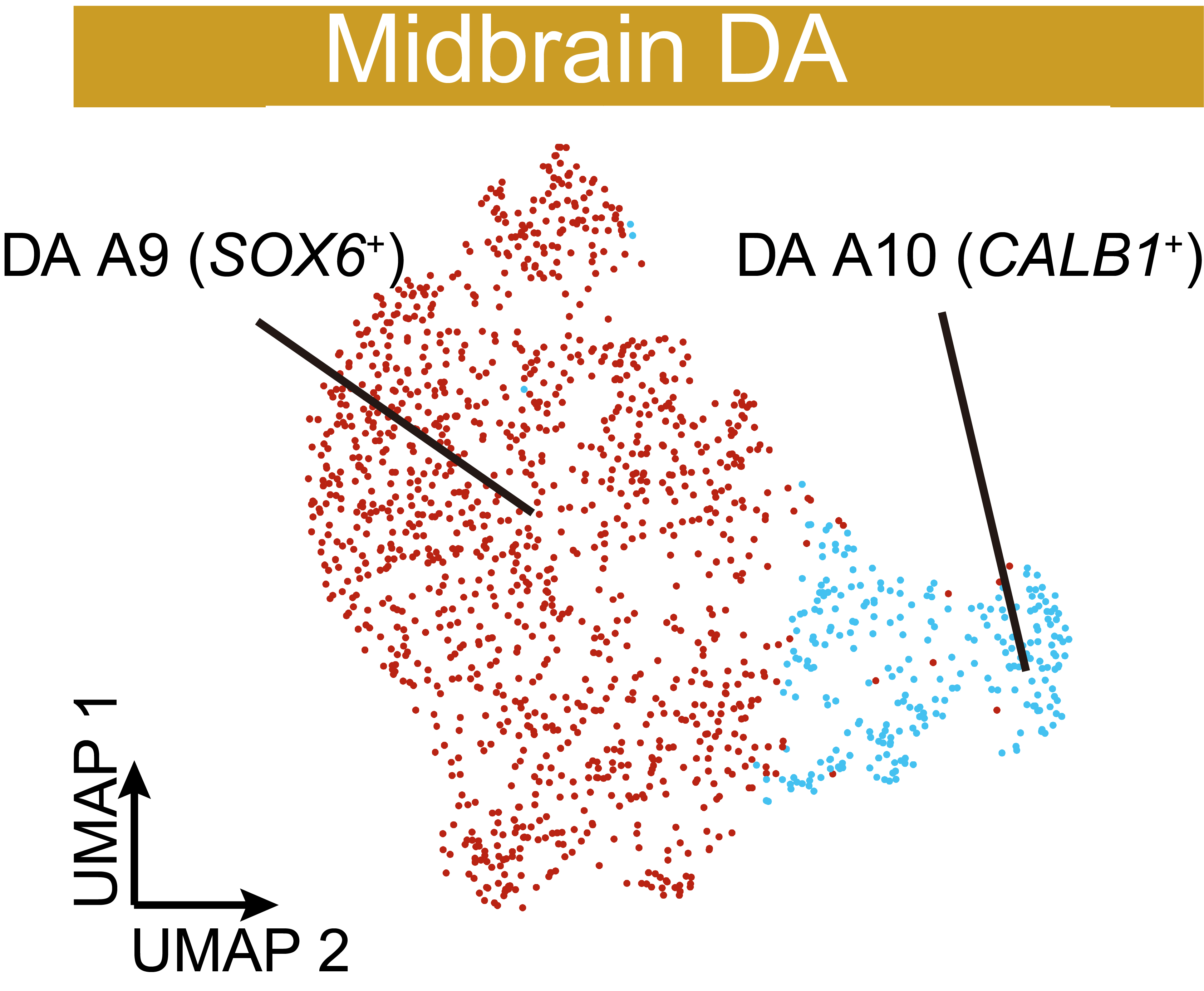
UMAP visualization of reclustered dopaminergic neurons in the midbrain. Two clusters of cells were defined based on the typical expression of SOX6 and CALB1.
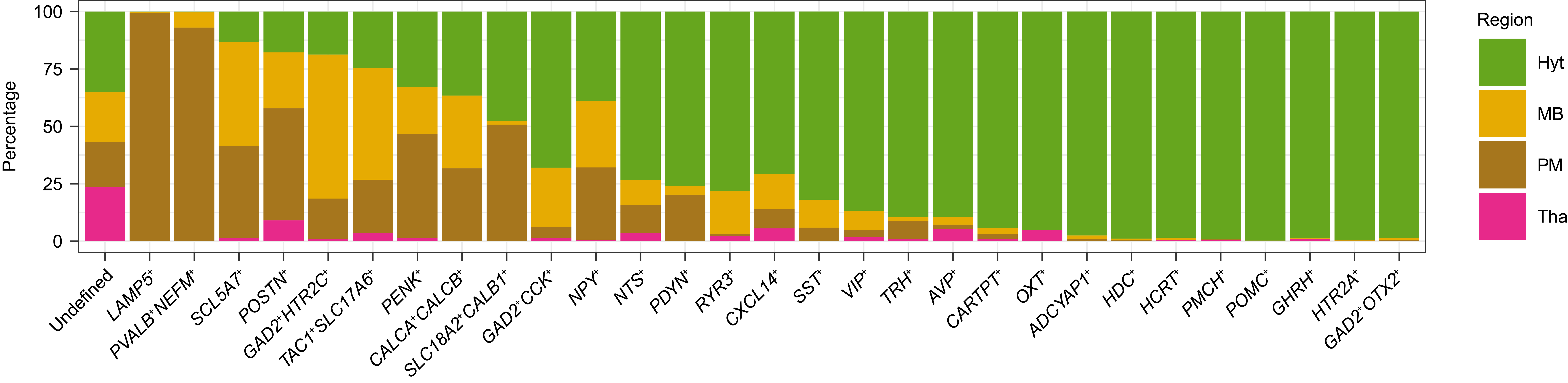
Stacked bar plot showing the percentage of splatter neuron subtypes identified in the different regions of the brain indicated in the right-hand side legend.

Left: Genome browser tracks showing the normalized aggregate peaks signal associated to TSS at the
KIT locus for MB-derived In in the monkey thalamus (top four rows, highlighted in pink) and
midbrain (bottom four rows, highlighted in orange).
Right: Genome browser tracks showing the normalized aggregate peaks signal associated to TSS at the
GFAP locus for Ast1 from the prefrontal cortex. The association between peaks and genes is
represented by the color scale. The homologous sites with humans as well as the distribution of DAREs in
the human genome are displayed in the lower part of each track plot.
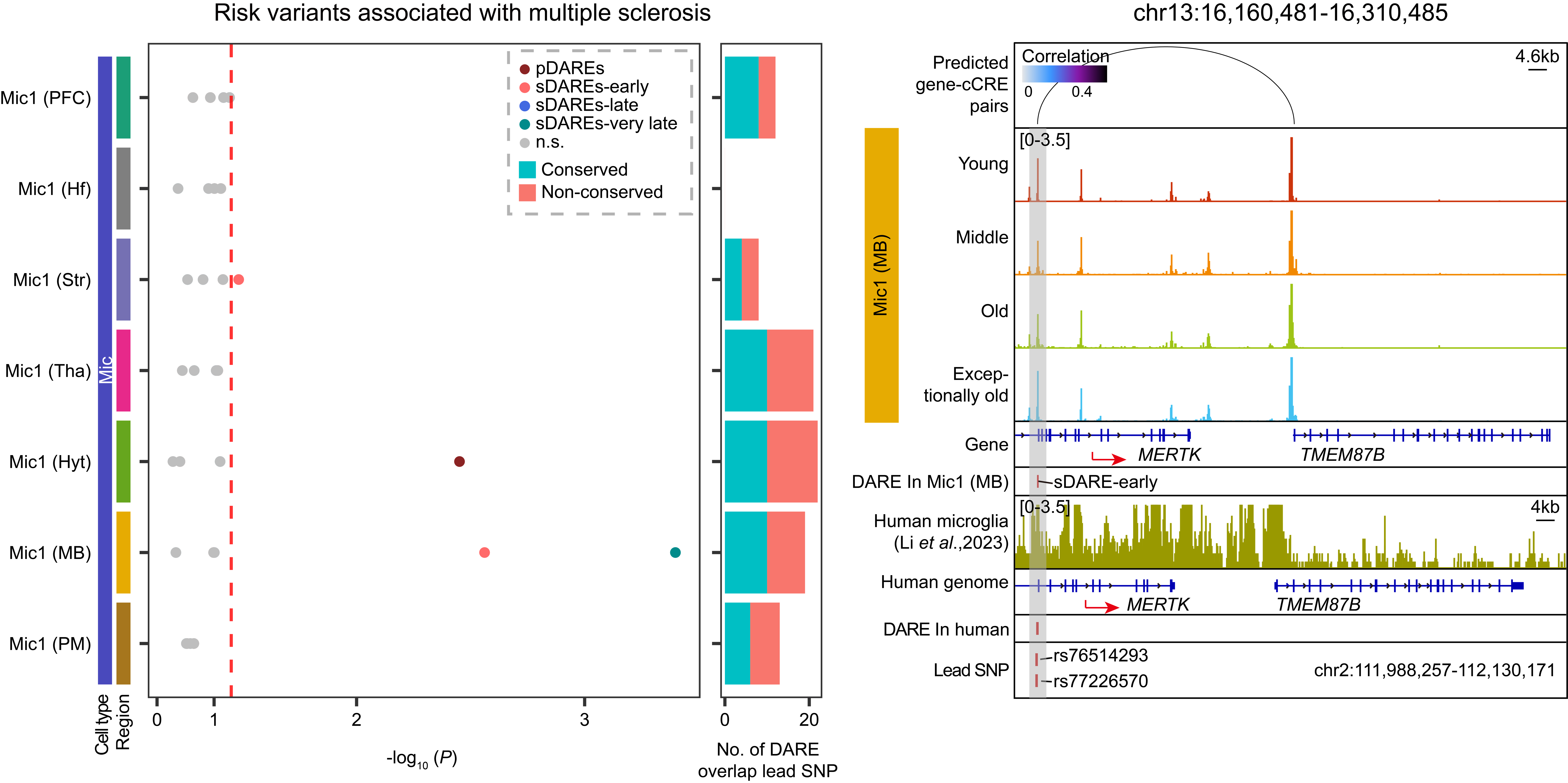
Left: Bubble plot showing the significant association of microglial DAREs with multiple sclerosis traits
in different microglial populations from the monkey brain tissues indicated on the left-hand side legend.
Dots are colored according to the type of DARE. The split bar plot indicates the number of DAREs
overlapping with lead SNPs associated with multiple sclerosis and colored according to their conservation
between human and monkey.
Right: Genome browser tracks showing the normalized aggregate peaks signal associated to TSS at the
TMEM87B locus for microglia cells from the midbrain. The association between peaks and genes is
represented by the color scale. The homologous sites with humans as well as the distribution of DAREs in
the human genome are displayed in the lower part of the track plot.
Explanation Notes
In the telencephalon, PFC Ex neurons (SLC17A7+) could be further subdivided into layer-specific subtypes including L2/3-IT (intratelencephalic; PDZD2+), L4/5-IT (RORB+), L5/6-IT-Car3 (claustrum-like; RGS12+), L5-IT (TSHZ2+), L5-ET (extratelencephalic; ADRA1A+), L5/6-NP (near-projecting; HTR2C+), L6-CT (corticothalamic; SEMA3E+) and L6-IT (ITGA8+)1,2. Hippocampal DG Ex neurons could be subclassified into BDNF+ and SYT10+.Among these, we observed a fraction of immature dentate granule cells (imGCs; PROX1+DCX+) indicative of adult neurogenesis, those being more enriched in SYT10+ neurons (see website). Hippocampal non-DG Ex neurons clustered according to their subanatomical location including the CA1/SUB (cornu ammonis subfields 1 and subiculum; PID1+), CA2/3 (cornu ammonis subfields 2 and 3; NRIP3+), and entorhinal cortex (EC) that was subdivided into upper layer (L2, CUX2+ RXFP1+; L3-5, TLL1+ PCP4+) and deep layer (L5/6, TLE4+ CPNE4+, L6, TLE4+ NXPH2+) neurons1. Additionally, the CA1/SUB and CA2/3 subpopulations could be further subdivided according to the superficial-deep axis (CA1/SUB: superficial CCBE1+ and deep NDST4+; CA2/3: superficial KCTD4+ and deep ST18+). Among the Inh neurons, we identified subpopulations derived from the MGE (LHX6+), CGE (ADARB2+), and lateral ganglionic eminence (LGE; FOXP2+)5. MGE-derived Inh neurons were distributed in all telencephalic regions, CGE-derived Inh neurons were mainly In the PFC and hippocampal formation, and LGE-derived Inh neurons in the striatum. MGE-derived Inh neurons could be further subdivided into PVALB+ and SST+ in the PFC and hippocampal formation, as well as SST+, TAC3+ and CRABP1+ in the striatum. We also noticed a small cluster of MGE-derived UNC5B+ chandelier cells in the PFC. CGE-derived Inh neurons contained LAMP5+ and CNR1+. LGE-derived Inh neurons were composed of SPNs (MEIS2+) including subtypes expressing the D1-type (SPN-D1; TAC1+) or D2-type (SPN-D2; PENK+) dopamine receptor, and eccentric SPNs (RBM20+). SPN-D1 contained a cluster belonging to neurochemically unique domains in the accumbens and putamen (SPN-D1-NUDAP; RXFP1+). In addition, we identified granule cells from the islands of Calleja (ICj) in the ventral striatum (ICj Inh; CPNE4+)6. In the thalamus, we observed a cluster of Ex neurons (SLC17A6+) comprising RYR3+ and CBLN2+ subtypes. RYR3+ neurons correspond to cortex-projecting neurons distributed in the ventral lateral and ventral anterior thalamus7, while CBLN2 is highly expressed in motor-related nuclei8. We also noticed GAD2+ SST+ Inh neurons characteristic of the thalamic reticular nucleus9, and GAD2+ OTX2+ midbrain-derived Inh neurons in the thalamus and midbrain10. Likewise, there were A9 (SOX6+) and A10 (CALB1+) subtype dopaminergic (DA) neurons (TH+) in the midbrain11, and in the pons and medulla a cluster of Ex neurons co-expressing SLC17A6/7 known to be distributed in most of the nuclei in this region10. In the cerebellum, besides cerebellar granule cells, we observed Purkinje cells (PPP1R17+), MLI1/2 (LYPD6+; CALB1+), Purkinje layer Inh (PLI; KLHL1+) neurons and unipolar brush cells (UBC; EOMES+)12.
Accessions
CNP0004459
Contact
laiyiwei@genomics.cn; zhangxiao6@genomics.cn; laiguangyao@genomics.cn